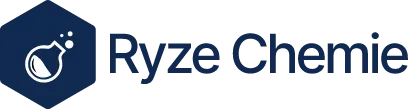
Stay up-to date on the
latest blogs. Join our
newsletter today!
This site is protected by reCAPTCHA and the Google Privacy Policy and Terms of Service apply.
How Does A Fermenter Work: All You Need To Know (2024)
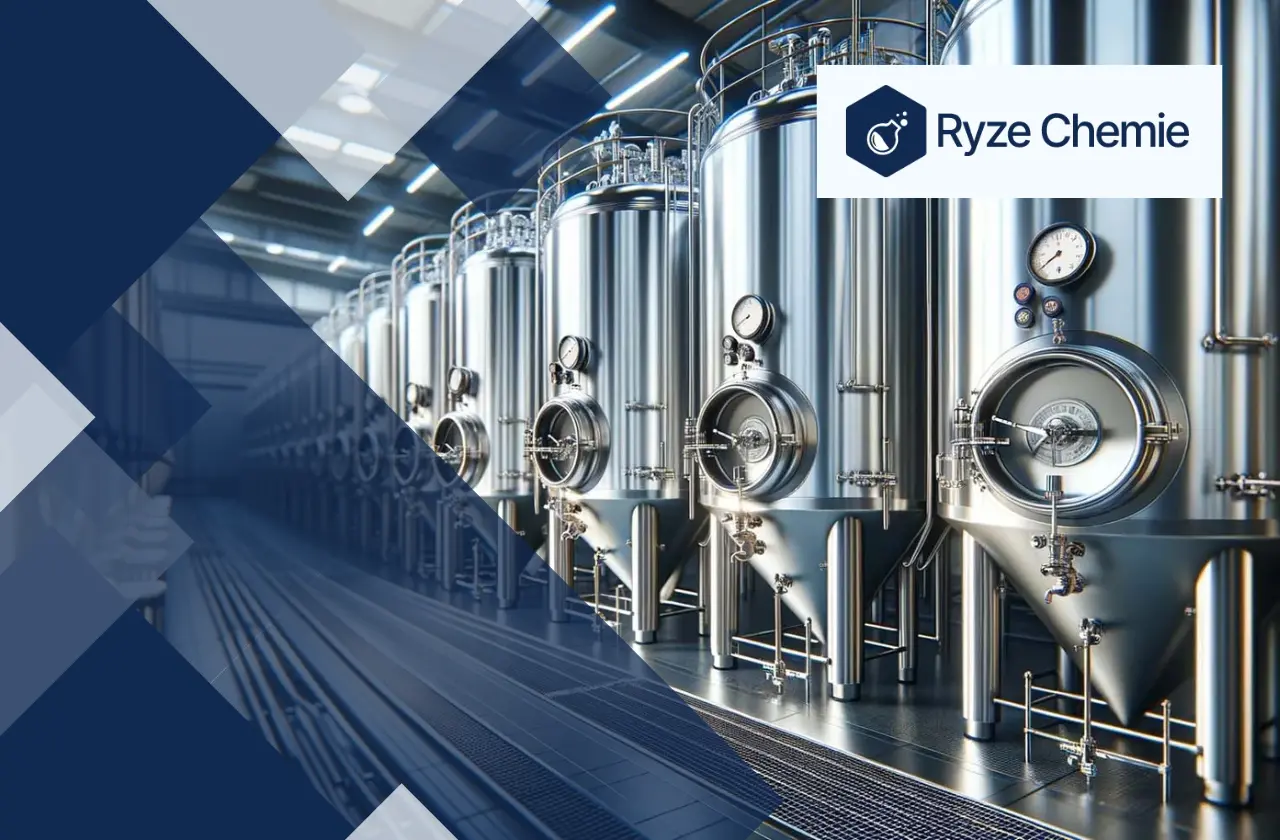
Written by Team Ryze Chemie
16 mins read · May 24, 2024
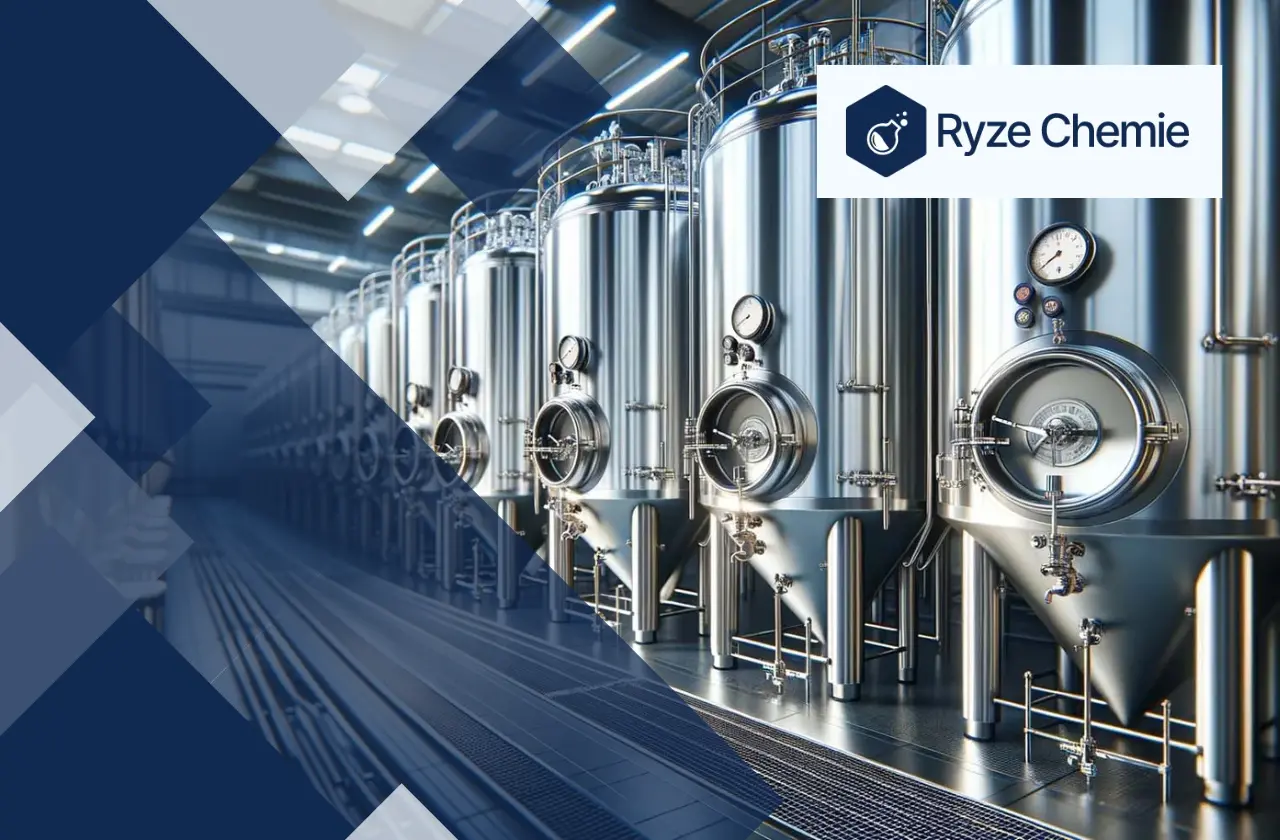
Fermenters are essential tools for laboratories and industrial processes. However, many lab personnel still struggle with understanding how fermenters actually work and how to optimize them for their specific applications.
This lack of understanding can lead to mistakes, inefficient processes, and even failed experiments. We understand your challenges. Our goal is to break down complex fermentation concepts into easy-to-understand terms.
In this article, we will explain the basics of fermenter operation, provide practical tips, and answer common questions to help you unlock the full potential of your fermentation processes.
Types of Fermenters
Fermenters come in many designs, each with a purpose. We will look at the most common ones used in our industry:
1) Stirred-Tank Fermenters
- Design: Stirred-tank fermenters are like big mixing bowls. They have a central shaft with paddles (impellers) that constantly stir the contents. This helps mix nutrients, oxygen, and the microorganisms.
- Mechanism: The stirring creates a uniform environment inside the fermenter. It ensures that every cell gets enough food and oxygen, which is crucial for growth and production.
- Advantages: They're versatile, easy to operate, and can handle large volumes. The design allows for good control over temperature and other conditions.
- Limitations: The moving parts can be prone to wear and tear. High energy costs are also a drawback due to the constant stirring.
2) Airlift Fermenters
- Design: Airlift fermenters use bubbles to mix things up. They have two main sections: a riser (where air is pumped) and a downcomer (where the liquid flows down).
- Mechanism: Bubbles create a circulating flow. Liquid rises with the air and then flows back down in the downcomer, creating a continuous loop.
- Advantages: Lower energy use compared to stirred tanks. They're gentler on cells, making them suitable for sensitive cultures.
- Limitations: Mixing efficiency can be lower than in stirred tanks, especially for thick liquids.
3) Bubble Column Fermenters
- Design: These are simple, vertical columns with a gas distributor at the bottom. Air is pumped through the distributor, creating a stream of bubbles.
- Mechanism: Bubbles rising through the liquid create mixing. This design relies on the natural flow patterns created by the bubbles.
- Advantages: Simple construction, low cost, and minimal moving parts mean less maintenance.
- Limitations: Mixing can be less efficient than other types, limiting their use for certain processes.
4) Other Specialized Fermenters
- Packed-Bed Fermenters: These have a solid packing material (like beads) on which the microorganisms grow. They're used for specific applications like ethanol production or wastewater treatment.
- Photobioreactors: These are designed for light-loving microorganisms like algae. They have transparent surfaces to maximize light exposure. They're used for growing algae for biofuels, food, or other products.
Having explored the different types, it's crucial to understand the key components that contribute to a fermenter's operation.
Key Components of a Fermenter
Every fermenter is equipped with essential components that play a vital role in maintaining the controlled environment necessary for successful fermentation:
1) The Vessel:
The vessel is the container where the fermentation process takes place.
- Materials: Stainless steel is the most common material for its durability and resistance to corrosion. Glass vessels are used for smaller, laboratory-scale fermenters, allowing for easy observation.
- Construction: Vessels are typically cylindrical with a domed top and bottom. They are designed to withstand pressure and maintain a sterile environment.
- Sterilization: Before each use, vessels are sterilized using methods like steam sterilization (autoclaving) or chemical sterilization (e.g., with peracetic acid) to eliminate unwanted microorganisms.
2) Agitator/Impeller:
Agitators ensure the contents of the fermenter are well mixed.
- Types: Common types include Rushton turbines (flat blades), marine propellers, and pitched-blade turbines. Each design offers different mixing characteristics.
- Function: Agitators prevent the settling of cells, distribute nutrients evenly, and help maintain a consistent temperature. They also promote oxygen transfer, which is vital for the growth of many microorganisms.
- Speed Control: Agitator speed is carefully controlled. Too slow, and the contents won't mix properly. Too fast, and it can damage cells.
3) Sparger:
Spargers introduce air or other gasses into the fermenter.
- Types: Porous spargers create a stream of small bubbles, while orifice spargers produce larger bubbles.
- Function: Spargers primarily supply oxygen for aerobic fermentation processes. The size and distribution of bubbles influence oxygen transfer efficiency.
- Oxygen Transfer: The rate of oxygen transfer is critical. Too little oxygen can limit growth, while excess oxygen can lead to foaming.
4) Baffles:
Baffles are vertical plates attached to the inner walls of the fermenter.
- Purpose: They disrupt the circular flow caused by agitation, preventing a vortex. This improves mixing efficiency and helps the sparger distribute oxygen evenly.
- Design: Baffle design considers the number, width, and placement for optimal performance in a specific fermenter.
5) Cooling/Heating System:
Precise temperature control is essential for optimal microbial growth and product formation.
- Importance: Many microorganisms have a narrow temperature range for optimal growth. Deviations can reduce productivity or even kill the culture.
- Mechanisms: Commonly used methods include water jackets (surrounding the vessel) or internal coils through which hot or cold water circulates.
6) Sensors and Probes:
Sensors provide real-time information about conditions inside the fermenter.
- Types: pH probes measure acidity, dissolved oxygen probes monitor oxygen levels, and temperature probes track temperature changes.
- Importance: These measurements allow for precise control and optimization of the fermentation process. Deviations from desired values can trigger alarms or automated adjustments.
With knowledge of the individual parts, we can now piece together how the entire fermentation process unfolds.
How Does A Fermenter Work
The fermentation process encompasses a series of distinct stages, each contributing to the transformation of raw materials into valuable products:
1) Inoculation:
Inoculation is like planting a seed for your experiment. It involves preparing a seed culture - basically, a small batch of the microorganism you want to use. We then transfer this culture to a larger vessel for fermentation. The key here is to be super careful to avoid any contamination, as unwanted microbes can mess up your results. This is where aseptic techniques come in handy - think sterile tools and a clean working environment.
2) Growth Phases:
Once your microbes are comfy in their new home, they go through different growth phases, each with its own quirks.
- Lag Phase: Think of this as your microbes' warm-up session. They're adjusting to their environment and not growing much.
- Exponential Phase: Now we're talking! Microbes multiply rapidly, doubling their numbers in a short time. This is where the magic happens.
- Stationary Phase: Party's over! Nutrients start to run out, and growth slows down. Some microbes might even start to die off.
- Death Phase: Things get grim as the number of dying microbes outpaces new growth.
Understanding these phases helps you figure out when to harvest your product or adjust conditions for optimal results.
3) Aeration and Oxygen Transfer:
Oxygen is like fuel for many microbes, especially in aerobic fermentation processes. To keep your microbes happy and productive, we need to ensure they get enough oxygen. This involves stirring or shaking the fermentation vessel and sometimes even pumping air or oxygen into it. It's like giving your microbes a breath of fresh air!
4) pH Control:
Microbes are picky about pH levels, and they perform best within a specific range. If they are too acidic or too alkaline, they might not grow or produce the desired products. We keep things balanced by adding acids or bases, acting as a buffer to maintain the ideal pH throughout the fermentation process. Think of it as creating a comfortable zone for your microbes.
5) Nutrient Feeding:
Just like us, microbes need food to grow and thrive. In batch fermentation, we add all the nutrients at the beginning. But sometimes, we feed nutrients continuously (continuous process) or at specific intervals (fed-batch process) to optimize growth and product formation.
6) Foam Control:
Foaming can be a real headache in fermentation. It can clog up equipment, reduce oxygen transfer, and even cause contamination. But don't worry; we have a few tricks up our sleeves. We use mechanical methods like foam breakers or chemical antifoams to keep the foam in check.
To ensure the fermentation process proceeds optimally, continuous monitoring and precise control are paramount.
Monitoring and Control Systems
Monitoring and control systems are the unsung heroes of laboratory work. They ensure safety, accuracy, and efficiency in your daily experiments. Let's break down how these systems work, why they matter, and how you can use them to your advantage:
1) Data Acquisition: The Foundation of Control
Imagine your lab experiments as a recipe. Data acquisition is like measuring the ingredients. You need the right amounts for the desired outcome.
- Sensors: These are the measuring cups of your lab. They detect temperature, pressure, flow rates, and other important parameters.
- Data Loggers: These are your recipe books. They store the sensor measurements over time.
- Accurate Data: Just like a good recipe, accurate data is essential. It ensures your experiments are repeatable and reliable.
Why it Matters:
- Safety: Sensors can detect dangerous conditions (like a gas leak) and trigger alarms.
- Efficiency: Data loggers track how your experiments perform, helping you optimize processes.
- Quality Control: Accurate data ensures your results meet high standards.
2) Feedback Control Loops: The Brain of the Operation
Feedback control loops are like the thermostat in your home. They maintain desired conditions by adjusting a process based on sensor data.
- PID Controllers: These are the brains of the loop. They use a mathematical formula to determine the best way to adjust your process.
- Setpoints: This is your target temperature – the desired condition you want to maintain.
- Tuning Strategies: Like adjusting a thermostat, you need to fine-tune PID controllers for optimal performance.
Why it Matters:
- Stability: Feedback control keeps your experiments running smoothly, even with disturbances.
- Precision: You can maintain very specific conditions, which are essential for many chemical reactions.
- Automation: Once set up, feedback control frees you up to focus on other tasks.
3) Automation and Supervisory Control: The Lab of the Future
Automation is like having a robot assistant in your lab. It takes care of repetitive tasks and monitors complex processes.
- SCADA Systems: These are the command centers for automation. They collect data from many sensors and control various equipment.
- Benefits: Increased productivity, improved safety, reduced human error.
- Challenges: It requires upfront investment and needs skilled personnel to set up and maintain it.
Why it Matters:
- Efficiency: Automate routine tasks to free up your time for more important work.
- Safety: SCADA systems can monitor hazardous processes and alert you to problems.
- Innovation: Embrace automation to stay at the forefront of modern laboratory practices.
Following fermentation, additional steps are required to obtain the final, refined product.
Downstream Processing
This final stage involves the separation, purification, and preparation of the desired product for its intended use:
1) Cell and Biomass Separation:
The Challenge: Your chemical reaction may be done, but now you have a messy soup of cells or other biological materials mixed in with your desired product. We need to get rid of these!
The Tools: Centrifugation and filtration are our workhorses here.
- Centrifugation: This is like a super-fast spin cycle on your washing machine. It uses centrifugal force to separate components by density. Heavier cells and solids are pulled to the bottom, leaving a clearer liquid on top.
- Filtration: This is more like using a coffee filter. We pass the mixture through a porous material. The liquid passes through, but larger cells and solids get trapped.
Pro Tip: The choice between centrifugation and filtration depends on the size of the particles and the volume of your mixture.
2) Product Purification:
The Challenge: We've removed the big stuff, but our product is still mixed with other chemicals. Time for some serious refining!
The Tools: Chromatography and extraction are our go-to methods here.
- Chromatography: This is like a race track for molecules. Different chemicals move at different speeds through a special material. We can collect our desired product as it reaches the finish line.
- Extraction: This is like dissolving sugar in water but in reverse. We use solvents to selectively pull out our desired product, leaving the impurities behind.
Pro Tip: There are many types of chromatography and extraction. Choosing the right one is key to getting a highly pure product.
3) Sterile and Aseptic Processing:
The Challenge: In some cases, like pharmaceuticals or certain biological products, contamination is not just an inconvenience; it's a major problem. We need to maintain sterile and aseptic conditions to ensure product safety and efficacy.
What This Means:
- Sterile: Completely free from all living microorganisms.
- Aseptic: Free from harmful contaminants (though some non-harmful microbes may be present).
How to Do It:
- Clean Work Areas: Thoroughly disinfect surfaces and equipment.
- Filtered Air: Use high-efficiency air filters to remove particles and microbes.
- Sterile Equipment: Autoclave or use other sterilization methods for tools and containers.
- Protective Clothing: Wear gloves, masks, and gowns to prevent contamination.
Pro Tip: Aseptic processing is easier to achieve than true sterility, but it's still essential for many high-value products.
Safety is of utmost importance when working with fermenters, and we must always be vigilant.
Safety Considerations
Working with fermenters entails inherent risks that we must be aware of. We will address these potential hazards and discuss how to mitigate them to ensure a safe working environment:
1) Sterilization and Containment
Why It Matters:
- Preventing contamination: A stray microbe or chemical residue can ruin an experiment, impacting results and potentially causing harm.
- Ensuring accuracy: Clean equipment and controlled environments lead to reliable data.
What to Do:
- Autoclave equipment: This uses high-pressure steam to kill most microorganisms.
- Use sterile technique: This involves working in a way that minimizes the introduction of unwanted organisms.
- Work in biosafety cabinets or fume hoods: These provide physical barriers to protect you and your work.
- Dispose of waste properly: Follow your lab's specific protocols for biological, chemical, and general waste disposal.
2) Pressure Relief Systems and Safety Interlocks
Why It Matters:
- Preventing explosions: Chemical reactions can generate pressure. Safety systems prevent dangerous buildups.
- Avoiding equipment damage: Overpressure can ruin expensive tools.
- Protecting personnel: Unexpected releases can cause injury.
What to Do:
- Know your equipment: Understand how pressure relief valves and safety interlocks work on your specific apparatus.
- Check regularly: Make sure these safety features are in good working order.
- Don't override: Never tamper with or disable safety systems.
3) Handling Hazardous Chemicals and Biological Materials
Why It Matters:
- Protecting yourself: Many chemicals are corrosive, toxic, or flammable. Biological materials can carry diseases.
- Protecting the environment: Improper disposal can contaminate water or soil.
What to Do:
- Read safety data sheets (SDS): These provide detailed information about the hazards of each chemical.
- Use appropriate PPE: This may include gloves, lab coats, eye protection, and respirators.
- Work in designated areas: Use fume hoods for volatile chemicals and biosafety cabinets for biological work.
- Store chemicals properly: This includes labeling containers clearly and segregating incompatible chemicals.
- Follow disposal protocols: Your lab will have specific procedures for safely disposing of hazardous materials.
4) Personal Protective Equipment (PPE)
Why It Matters:
- Your first line of defense: PPE acts as a barrier between you and potential hazards.
- Staying comfortable: The right PPE helps you work safely without distraction.
What to Do:
- Gloves: Choose the right type for the chemicals you are working with.
- Lab coat: Protects your skin and clothes from splashes and spills.
- Eye protection: Safety glasses or goggles are essential when working with chemicals or glassware.
- Respirators: These may be required to work with volatile chemicals or infectious agents.
Despite our best efforts, issues may occasionally arise. Let's explore some common problems that can occur within fermenters.
Troubleshooting Common Fermenter Issues
We will examine several problems that can arise during fermentation, along with effective strategies for resolving them:
1) Contamination:
What to Look For:
- Cloudy Broth: Your fermentation broth looks murky or changes color unexpectedly. This can be a sign of bacterial or fungal growth.
- Off Odors: A funky smell (think vinegar or rotten eggs) can indicate unwanted microbes.
- Slow or Stalled Fermentation: If your process isn't progressing as expected, contamination could be the culprit.
How to Prevent It:
- Sterilize Everything: Autoclave your fermenter, tubing, and any tools you use.
- Use Proper Technique: Be meticulous when transferring cultures and adding ingredients. Work in a clean environment like a laminar flow hood.
- Monitor Regularly: Keep an eye on your fermentation broth and take samples for microscopic examination if you suspect something's wrong.
2) Oxygen Issues, Foam, and pH Ups and Downs
Oxygen Trouble:
- Poor Growth: If your microbes need oxygen, insufficient aeration can stunt their growth.
- Check Aeration: Make sure your air supply is working correctly, and adjust the flow rate as needed.
Foam Frenzy:
- Messy Overflow: Foam can clog filters and make a mess.
- Antifoams to the Rescue: Add a few drops of antifoam agent to your fermenter to keep things under control.
pH Problems:
- Unhappy Microbes: The wrong pH can stress your microbes and affect their performance.
- Regular Testing is Key: Monitor pH regularly and adjust it with acid or base solutions as needed.
3) Low Yields and Slow Growth:
Possible Causes:
- Nutrient Shortages: Your microbes might be running out of food.
- Temperature Troubles: Too hot or too cold can slow down growth.
- Inhibitors: Some byproducts of fermentation can inhibit growth.
What to Do:
- Check Your Recipe: Make sure your media has all the nutrients your microbes need.
- Temperature Check: Verify that your fermenter is maintaining the correct temperature.
- Consider a Fed-Batch: Gradually add nutrients to keep the microbes happy and productive.
4) Sensor Calibration and Maintenance:
- Inaccurate Readings: Sensors can drift over time, leading to unreliable data.
- Regular Calibration: Calibrate your sensors according to the manufacturer's instructions.
- Cleanliness is Key: Keep your sensors clean and free of buildup.
Bonus Tip: Always have spare sensors on hand in case one fails.
Conclusion
Fermenters are like mini-factories, transforming simple ingredients into valuable products. They control the environment perfectly, making sure microbes thrive and work efficiently. Scientists and industries alike depend on fermenters for breakthroughs in medicine, food, and biotechnology.
Understanding how fermenters work opens doors to exciting possibilities, from developing new drugs to creating eco-friendly materials. With a solid grasp of this process, laboratory personnel like yourselves can push boundaries and make a real difference.
Latest Blogs